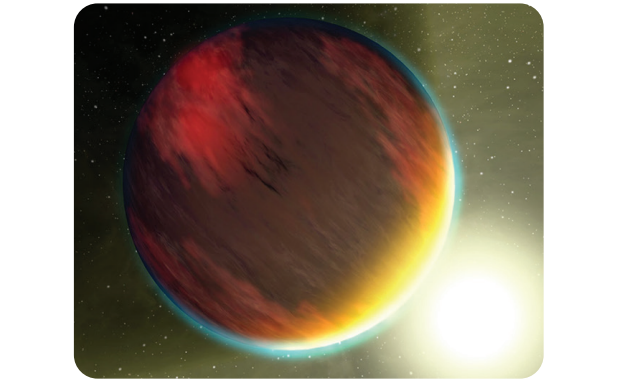
Figure 1 Artist‘s concept of an exoplanet. Credit: NASA
INTRODUCTION
An exoplanet (or extrasolar planet) is a planet, which orbits a different star than our Sun. Planets which are found outside of the solar system but are not gravitationally bound to any star are called rough (or interstellar, nomad etc.) planets these will not be discussed in this text. Over the 30 years since the first detection of an exoplane t in 1988 (as of August 1 2018), there are 3815 confirmed exoplanets, which are organized into 2853 systems. Most of these exoplanets (over 2000) were discovered by the Kepler space telescope, whose launch in 2009 represented a major breakthrough in the hu nt for new exoplanets. It is now believed that presence of planets in other stellar systems is a commonplace phenomenon. There are even estimates that statistically one in five Sun like stars harbors an Earth like exoplanet in the habitable zone (that is i n the range of distances from the central star where liquid water can exist, given sufficient atmospheric pressure). Physical characteristics of the so far detected exoplanets, however, range over very wide intervals: starting with light weight stony plane ts with masses comparable to the mass of the Moon and the so called “super Earths” (with masses 2 to 10 Earth masses) we can go all the way up to very massive gas giants, which are ten times as massive as Jupiter. From ultra short orbital periods within ho urs to planets which take thousands of years to orbit their star. From planets with surface temperature reaching thousand kelvins going down to habitable planets and icy worlds. More exotic examples include planets orbiting a pulsar, binary or even multip le star systems. Explanation of Figure 1 Artist‘s concept of an exoplanet. Galactic Neighbourhood this variability of properties exhibited by the exoplanets discovered to date is a challenge for this variability of properties exhibited by the exoplanets discovered to date is a challenge for astrophysicists who focus on the dynamics (origin and evolution) of planetary systems. Especially astrophysicists who focus on the dynamics (origin and evolution) of planetary systems. Especially interesting are of course thinteresting are of course the exoplanets, whose orbits lie in the habitable zone and thus may provide e exoplanets, whose orbits lie in the habitable zone and thus may provide conditions suitable for life. By studying the atmospheric spectra of these exoplanets, one may conditions suitable for life. By studying the atmospheric spectra of these exoplanets, one may uncover (such as molecular oxygen or methane), which are typical for presence of lifefouncover (such as molecular oxygen or methane), which are typical for presence of lifeforms. rms. AstrobiologyAstrobiology is a growing scientific field concerned with general questions such as formation and is a growing scientific field concerned with general questions such as formation and detection of life on exoplanets.detection of life on exoplanets.
Content
After a brief account of history of the subject, we shall describe some of the methods which are After a brief account of history of the subject, we shall describe some of the methods which are nowadays used nowadays used for detection of exoplanets. For each method, we will specify physical quantities for detection of exoplanets. For each method, we will specify physical quantities which can be measured (observables) and summarize its results. In the practical part, we will offer a which can be measured (observables) and summarize its results. In the practical part, we will offer a couple exercises ranging from very simple questions to more complicated couple exercises ranging from very simple questions to more complicated problems and activities.problems and activities.
THEORETICAL PART FOR THE TEACHER
Astronomers introduced the following systematic convention for designating exoplanets: a lower case letter of Latin alphabet (starting with “b”) is appended to the designation of the parent star. The letter indicates the order in which, for the given host star, the exoplanet was discovered. For instance, HIP 75458b denotes the first exoplanet which was discovered orbiting the star with designation HIP 75458. On the other hand, 55 Cancri e is the fourth exoplanet detected in the system hosted by the star 55 Cancri.
History
The existence of planets outside of our solar system was a subject of speculations among astronomers, philosophers and various science fiction authors over past centuries. It was Giordano Bruno (that is still deep within the period dominated by heliocentri c thoughts) who started playing with the idea that stars are, in fact, objects similar to our Sun and as such they could host planetary with the idea that stars are, in fact, objects similar to our Sun and as such they could host planetary systems. Propositions of this sort continued to pour in over the years to follow. In his systems. Propositions of this sort continued to pour in over the years to follow. In his Principia,Principia, Isaac Newton, for example, puts the solar system essentially on equal footing with planetary Isaac Newton, for example, puts the solar system essentially on equal footing with planetary systems orbiting different stars. Detection of exoplanets was attempted by many astronomers using systems orbiting different stars. Detection of exoplanets was attempted by many astronomers using various methods, but to no avail. The closest they could get wasvarious methods, but to no avail. The closest they could get was the discovery of protoplanetary the discovery of protoplanetary disc around the star Beta Pictoris in 1983. The longdisc around the star Beta Pictoris in 1983. The long--expected breakthrough finally happened in expected breakthrough finally happened in 1988, when an exoplanet was discovered orbiting the star Gamma Cephei by the Canadian 1988, when an exoplanet was discovered orbiting the star Gamma Cephei by the Canadian astronomers B. Campbell, G. Walker and S. astronomers B. Campbell, G. Walker and S. Yang (although it was fully confirmed only in 2003). Yang (although it was fully confirmed only in 2003). This exoplanet, which was originally detected using the method of radial velocities, falls into the This exoplanet, which was originally detected using the method of radial velocities, falls into the category of „hot Jupiters“ (see below for explanation). This was followed by the discovery of the category of „hot Jupiters“ (see below for explanation). This was followed by the discovery of the planeplanetary system around the pulsar PSR 1257+12 in 1992 and also the first exoplanet orbiting a tary system around the pulsar PSR 1257+12 in 1992 and also the first exoplanet orbiting a main sequence star (51 Pegasi) in 1995. Contrary to the case of Gamma Cephei b, both of these main sequence star (51 Pegasi) in 1995. Contrary to the case of Gamma Cephei b, both of these exoplanets were confirmed almost immediately, so it is not clear which oexoplanets were confirmed almost immediately, so it is not clear which one of these three ne of these three discoveries should be considered the first detection of an exoplanet. The number of discoveries discoveries should be considered the first detection of an exoplanet. The number of discoveries started mounting very quickly around the year 2000. Below we show a summary of the most started mounting very quickly around the year 2000. Below we show a summary of the most important milestones.important milestones.
1988: first detection of an ex1988: first detection of an exoplanet, which was later confirmed (Gamma Cephei b)
1992: first exoplanet orbiting a pulsar (PSR 1257+12b)
1996: first exoplanet orbiting a main1996: first exoplanet orbiting a main--sequence star (51 Pegasi b)
1999: first transiting exoplanet (HD 209458b), first multiplanetary system (U1999: first transiting exoplanet (HD 209458b)
2001: first exoplanet orbiting in habitable zone (HD 28185b), first measurement of 2001: first exoplanet orbiting in habitable zone (HD 28185b)
2005: first direct observation of en exoplanet (HD 209458b, by Spitzer space telescope in IR)
2006: launch of C2006: launch of CoRoT, first satellite specialized on detecting exoplanet (using the method oRoT, first satellite specialized on detecting exoplanet (using the method of transits)
2009: launch of Kepler space telescope, which continuously measures brightness of roughly 2009: launch of Kepler space telescope, which continuously measures brightness of roughly 15000 stars and searches for signs of exoplanets; first terrestrial exoplane15000 stars and searches for signs of exoplanets; first terrestrial exoplanet discovered (CoRoTt discovered (CoRoT--7b)
2014: first stony Earth2014: first stony Earth--sized exoplanet orbiting in habitable zone (Keplersized exoplanet orbiting in habitable zone (Kepler--186f)
Methods of detection
The basic obstacle which makes it very difficult to observe exoplanets is that these are relatively small and at the same time very distant objects which can only be seen through the reflected light of they host star. This is further deteriorated by the fact that from the Earth, exoplanets are seen at very small angular distances from their host stars, so their light is completely washed out by the light coming directly from the host star. Even tough the observational equipment has already advanced
coming directly from the host star. Even tough the observational equipment has already advanced enough to enable direct imaging of a small number of exoplanets (which orbit at large enough enough to enable direct imaging of a small number of exoplanets (which orbit at large enough angular separation from their host stars)angular separation from their host stars), astronomers still have to rely on indirect methods in the , astronomers still have to rely on indirect methods in the large majority of cases. This is corroborated by the data shown on Fig. 2, which gives the number of large majority of cases. This is corroborated by the data shown on Fig. 2, which gives the number of exoplanets detected by specific methods over time. The following paragraphs will give a detaileexoplanets detected by specific methods over time. The following paragraphs will give a detailed d description of the two most common indirect methods of exoplanet detection: description of the two most common indirect methods of exoplanet detection: radialradial--velocity velocity methodmethod and and transit photometrytransit photometry. .
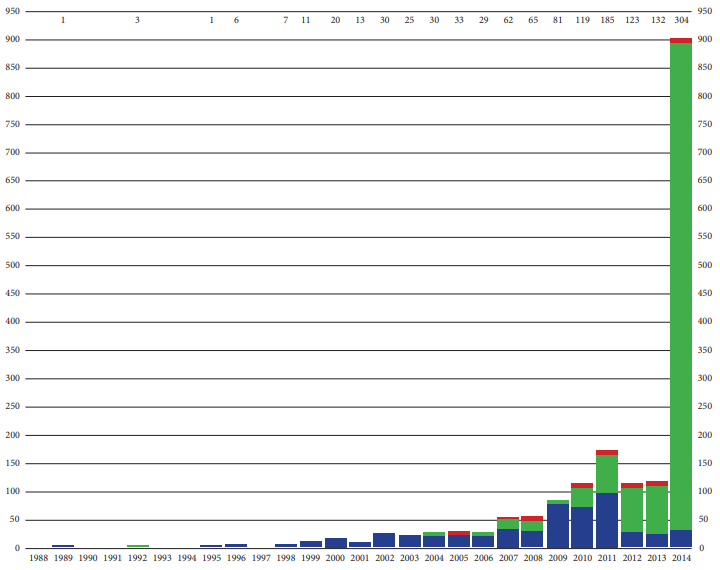
Figure 2 Number of exoplanets discovered over time by the method of their detection. Red: direct imaging, orange: microlensing, green: transit photometry, blue: radial velocity method. Source: Wikipedia (CC licence)
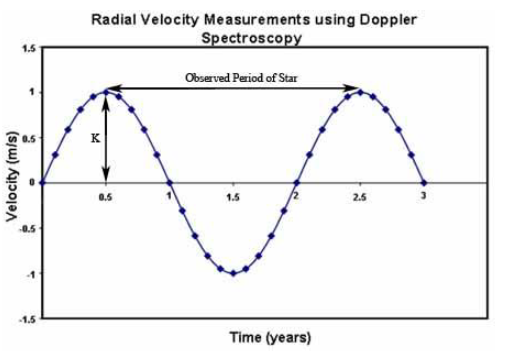
Figure 3: Plot of radial velocity of the host star as a function of time. Source: Wikipedia (CC licence)
Method of radial velocities
This method uses one of the basic principles of two body dynamics: relative to an inertial reference frame, smaller body does not orbit around the larger one, but both bodies orbit around their common center of mass. The presence of an exoplanet therefore causes that the host star follows a (very small) orbit around the center of mass of the system. From the point of view of an Earth based observer, this means that the radial component of the star’s velocity relative to the earth periodically changes (see Fig. 3). This manifests itself in the spectrum of the host star as a periodial(Doppler) of the absorption lines about their mean positions which, in principle, can be detected
(Doppler) of the absorption lines about their mean positions which, in principle, can be detected using a spectrometer. Magnitude of this shift depends on a number of factors. Fusing a spectrometer. Magnitude of this shift depends on a number of factors. First, the ratio of the irst, the ratio of the orbital speeds of the star and the exoplanet around their center of mass is equal to the ratio of the orbital speeds of the star and the exoplanet around their center of mass is equal to the ratio of the mass of the exoplanet to the mass of the star. Second, orbital speed of the exoplanet is inversely mass of the exoplanet to the mass of the star. Second, orbital speed of the exoplanet is inversely proportional to the squareproportional to the square--root root of the distance to the center of mass of the system. It therefore of the distance to the center of mass of the system. It therefore follows that the large the mass of the exoplanet and the closer it orbits to its parent star, the larger follows that the large the mass of the exoplanet and the closer it orbits to its parent star, the larger the orbital speed of the star around the center of mass. Typical values of the orbitathe orbital speed of the star around the center of mass. Typical values of the orbital speeds of l speeds of exoplanets are of the order of tens or hundreds of km/s. For the Sunexoplanets are of the order of tens or hundreds of km/s. For the Sun--like stars and Jupiterlike stars and Jupiter--sized sized exoplanets, this gives orbital speeds of the host star around the center of mass in the order of units exoplanets, this gives orbital speeds of the host star around the center of mass in the order of units of m/s. The amplitude of radial velocity anof m/s. The amplitude of radial velocity and hence the amplitude of the spectral line shift further d hence the amplitude of the spectral line shift further depend on the inclination of the orbital plane of the system relative to the line of sight: in the case depend on the inclination of the orbital plane of the system relative to the line of sight: in the case where the orbital plane is perpendicular to the line of sight, the projection of the orbital vewhere the orbital plane is perpendicular to the line of sight, the projection of the orbital velocity into locity into the radial direction is zero which renders the method useless. On the other hand, the method is the radial direction is zero which renders the method useless. On the other hand, the method is ideally suited to the cases when the line of sight lies in the orbital plane and the amplitude of the ideally suited to the cases when the line of sight lies in the orbital plane and the amplitude of the changes in radial velocity is therefore equal changes in radial velocity is therefore equal to the orbital speed. For the above considered case, we to the orbital speed. For the above considered case, we then obtain the amplitude of the Doppler shift of absorption line of the order 10then obtain the amplitude of the Doppler shift of absorption line of the order 10--5 nm. Our ability to 5 nm. Our ability to detect exoplanets using the radialdetect exoplanets using the radial--velocity method is also affected by the orbital period: large velocity method is also affected by the orbital period: large values values of the orbital period make it difficult to notice the small changes in radial velocity. Physical of the orbital period make it difficult to notice the small changes in radial velocity. Physical characteristics of the system, which can be directly measured using the method include the orbital characteristics of the system, which can be directly measured using the method include the orbital period and the amplitude of radial velocity. Assuming that we are able to estimate the mass of the host star, size of the orbit and also the effective mass M _p sin i (where i is the generally unknown inclination of the orbital plane) can be inferred by us ing the 3 rd Kepler’s law.
This is easily seen to be a consequence of the radial velocity method being most effective exactly in the cases of massive exoplanets orbiting close to the central star with short orbital period, as described above. The method therefore gives rise to a selection bias: were it not for other methods of exoplanet detection, we would conclude that typical planeta ry systems contain objects which exhibit fairly extreme characteristics compared to the planets orbiting the Sun. The most successful “hunters” of exoplanets include the spectrometers HARPS (High Accuracy Radial Velocity Planet Searcher) which is placed on the 3.6 m ESO telescope on La Silla and spectrometer HIRES (HIgh Resolution Echelle Spectrometer) which works with one of the Keck telescopes. Both instruments enable radial velocity amplitude measurement with accuracy around 1 m/s.
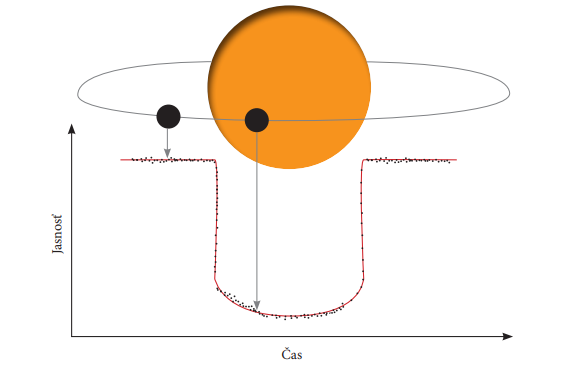
Figure 4 Transit of an exoplanet over the disk of its host star.
Transit photometry
On the other hand, the so called secondary minimum, which is due to the exoplanet being blocked by the star, is virtually absent it can, however, be measured by modern methods ). The moment when the brightness starts decreasing is usually called the first contact and it stops decreasing at the second contact. The brightness starts increasing again upon the third contact and regains its original value at the fourth contact. The decrease in brightness of the host start due to the transit i.e. the depth of the transit ) depends mainly on the ratio of the exoplanet radius to the radius of the host star. The closer this ratio is to 1 the larger part of the host star is eclipsed and the more pronounced is the decrease in brightness. To give one example, the transit of the Earth would cause a dip of about 0,0001 mag in the brightness of the Sun as measured by a distant observer (this corresponds to 0,008 % of Sun’s total brightness) brightness). As we have already mentioned, our ability to observe transits depends on the geometry of exoplanet’s orbit: the systems for which the angle between the line of sight and the orbital plane is too large the transits may not occur . The physical quantities which can be measured directly from the light curve include the orbital period and the ratio of t he radii of the host star and the exoplanet. Assuming that we are also able to estimate the mass of the host star, we can use the 3rd Kepler‘ s law to calculate the size of the orbit. Given that we can also measure the intervals between individual contacts, we can actually reconstruct the full goemetry of the system, that is we can infer the radii of both the host star and the exoplanet, together with the inclination angle. When combined with the radial velocity method (which gives the mass of the exoplanet) the transit photometry can therefore give information about the density of the exoplanet. This allows to determine its typ gas giant / terrestrial planet / icy planet /...
As it is apparent from Fig. 2 , the method of transit photometry yielded by far the most discoveries of new exoplanets, mostly courtesy of the Kepler space telescope, which itself accounts for 2 000 confirmed detections and about 10 000 candidates as of August 2018). Modern instruments can achieve accuracy which allows for detection of planets smaller than Earth. The tendency to produce selection bias of any kind is generally much lower for the transit photometry than for the radial velocity method. Besides the stony planets of terrestrial type, the method of transit photometry showed existence of exoplanets of the type not seen in the solar system, the so called super Earth e.g. CoRoT 7b). That is planets with masses greater than that of the Earth but at the same time much lower than the mass of Uranus or Neptune. Transit photometry is also the only method which can be successfully performed by amateur astronomers.