INTRODUCTION
Space… is big. Really big. You just won´t believe how vastly, hugely, mindbogglingly big it is.
Douglas Adams, The Hitchhiker’s Guide to the Galaxy
THE UNIVERSE IS INCONCEIVABLY BIG
The space in the Universe is big and, according to traditional human measures, it is difficult to imagine just the distances within the Solar System, not those between the stars or galaxies. Moreover, measuring the distances in the Universe is generally rather difficult and, as it has been explained in the previous chapters, we use various units in various scales in order to be able to work with “reasonably” big numbers. While on Earth and its surroundings, we manage with hundreds and thousands of kilometres; within the Solar System we use the astronomical unit as the most demonstrative one and beyond the boundaries of the Solar System we use the light years or parsecs and their multiples (kly, Mly, Gly, kpc, Mpc). Generally, the Universal space can be divided into interplanetary (within the Solar system or similar exoplanetary systems in the neighbourhood of other stars), interstellar and intergalactic.
A well-known German philosopher, Immanuel Kant (1724 – 1804), was not the only one who was filled with astonishment by the sky full of stars. Since ancient times people perceived enormous greatness (even though they could only hardly imagine it and since antiquity until today they have been repeatedly discovering that the Universe is much bigger than they had thought). They admired the aggregations of stars (today referred to as constellations) and they invented the whole pack of myths about their origin (when looking at Perseus, Cassiopeia or Orion constellations, we remember the heroes from ancient Greek mythology as well). Stars, obviously, are not evenly dispersed in the sky, but they form aggregations. Modern telescopes discovered that even the galaxies, thanks to their gravitational effect, tend to cluster into complex patterns and create groups, clusters of galaxies and long filaments extending to hundreds of millions of light years.
Distances in the Universe
Milky Way Galaxy
Our Sun and 200 billion of other stars form our Galaxy called Milky Way. If we could look at it from the distance of millions of light years, we would see that the stars are organized into a shape of a disc with more than 100 000 ly in diameter. We can find the similar structure in some other galaxies, e.g. M31 in Andromeda constellation. The distance of our Solar System from the centre of the Galaxy is approximately 30 000 ly (Fig. 1). The Solar System (and thus all of us) rotate around the Galaxy centre at an enormous speed of 230 km/s and one such orbit takes 240 million years.
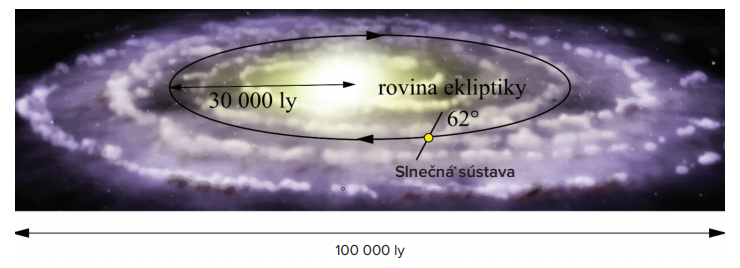
Fig. 1 Scheme with the dimensions of our Galaxy (Milky Way), the size of the Solar System does not correspond to the scale – it is larger to be visible; plane of ecliptics forms a 62° angle with the galactic plane (adapted from http://cococubed.asu.edu/pix_pages/astro101.shtml)
Likewise the stars, galaxies also group into bigger aggregations. Our Galaxy, together with the Great galaxy M31 in Andromeda, M33 Galaxy in Triangulum and thirty other smaller galaxies form an isolated system, so-called Local Group of galaxies. M31 Galaxy is approaching our Galaxy at a speed of 200 km/s and in approximately a billion of years it will come into collision with the Milky Way. Other well-known „members“ of our Local Group are irregular galaxies - Large and Small Magellanic Clouds, irregular dwarf galaxies some 200 000 ly distant from our Galaxy. Local Group is sometimes called Local Cluster of Galaxies (Fig.2), the biggest representatives within being our Galaxy and M31 Galaxy in Andromeda.
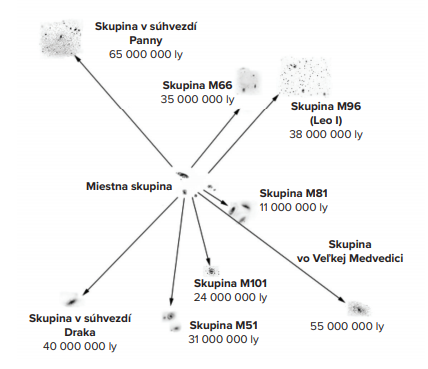
Fig. 2: Distances to some of the other galaxies in the „proximity“ of our Local Group (adapted from https://imagine.gsfc.nasa.gov)
Giant cluster of galaxies in Virgo constellation is approximately 60 000 000 ly away from our Milky Way. It forms the heart of the local supercluster of galaxies, sometimes called the supergalaxy. It comprises about 100 000 galaxies in the space of 100million of ly in dimension. To this day, we know tens of supergalaxies which are situated in the dispersion knots of galaxies (Fig.3 we can see that the dispersion structure resembles a network of fungi filaments).
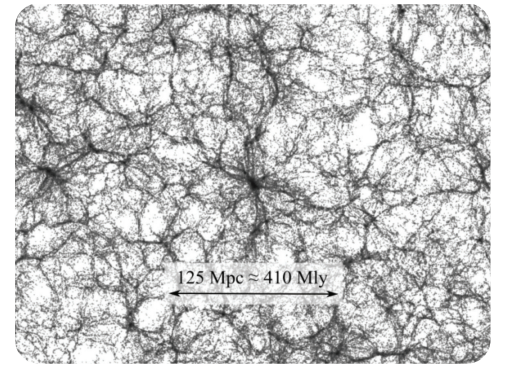
Fig. 3: Computer simulation of dispersion of galaxies in the Universe with the typical „filament structure“ (Source: The Millennium Simulation Project, https://wwwmpa.mpa-garching.mpg.de/galform/virgo/millennium/index.shtml)
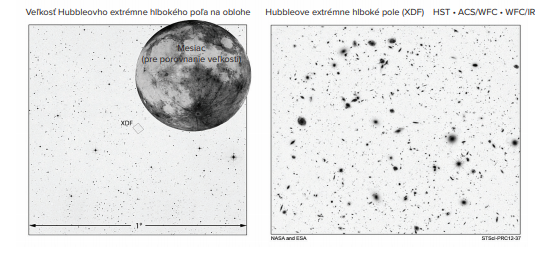
Fig. 4: Hubble Extreme Deep Field, view of the remotest areas of the Universe (Source: NASA, ESA, http://hubblesite.org/images/news/release/2012-37)
Smaller distances within the Solar System are measured by means of a radar or laser, thanks to what we know the Earth – Moon distance with an accuracy of a few millimetres. Parallax can be used for the distances up to 1 000 ly. Satellite Hipparchos, named after the famous ancient Greek astronomer, measured the parallaxes of 120 000 stars in the years 1989 – 1993. Ongoing measurement of Gaia satellite, launched in 2013, should determine the distances of approximately one billion of stars with great accuracy.
In order to determine greater distances, we use the principle that more remote stars appear less bright to us – from a generally same star situated in a double distance we capture four times less luminosity energy in the same area. However, we need to determine the luminosity of the star (i.e. how much energy it emits per second). Luminosity value for the Sun is huge: 4·1026 W (the power of nuclear power plant Temelín is 2·109 W). Object, whose brightness we know or are able to calculate, is termed a standard candle (Fig. 5).
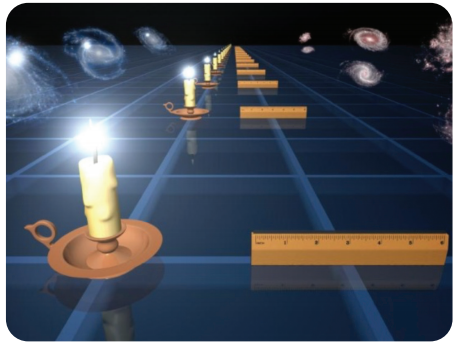
Fig. 5: Principle of the Standard Candle – the same candle further away shines less from our point of view, we capture less luminosity energy on the same surface of the telescope; we know the principle from the nightly observation of e.g. streetlights in the long street – the ones further away shine less for us (source: NASA, https://www.nasa.gov/mission_pages/galex/pia14095.html)
Cepheids and supernovas
Cepheids, pulsating variable stars, can be used as standard candles. The period of their changes of brightness in the sky, related to Cepheid luminosity, can be easily measured. If we measure the period of brightness pulsation, we can calculate the Cepheid luminosity. By comparing the real brightness and known luminosity we can determine their distance. Cepheids were first used to measure distances in 1912 by Henrietta Swan Leavitt. By means of Cepheids, Edwin Hubble discovered the expansion of Universe in 1929.
Supernovas of Ia type, observable to the distance of millions or even billions of light years, are another type of standard candles. They represent a final stage of a close binary star out of which one is a white dwarf and another a giant (or supergiant). The transfer of mass from the giant star to the white dwarf causes the dwarf to increase its weight and, if it exceeds the value of 1.4 of the weight of the Sun, a massive explosion occurs discharging in few seconds much more energy than hundreds billions of Suns. Cepheids and Ia supernovas are important part of the cosmic „distance ladder“ (Fig. 6).
Is the cosmic space infinite? We are not sure about the answer, but we suppose that the Universe has no boundaries (it is hard to imagine a sign „end of the Universe“ and what would be behind it) – so it must be either infinite or self-centred similarly to the spherical surface of the Earth, which we can circumnavigate or orbit. We are not able to observe the Universe as a whole, only its part, so-called observable Universe. Its boundary is determined by the finite speed of light – we can observe only such part of the Universe from which the light (or other electromagnetic radiation) has managed to reach us since the beginning of the existence of Universe (approximately 14 billion of years).
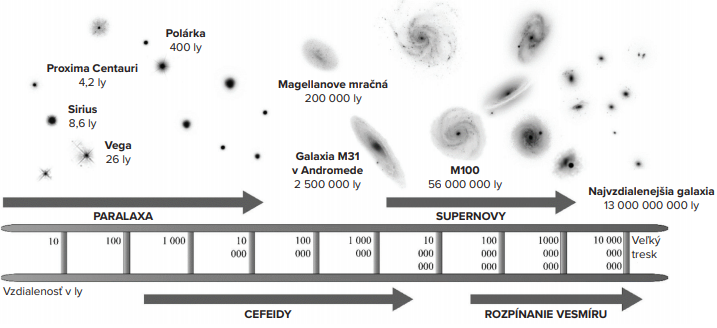
Fig. 6: We use various methods and techniques to determine the distances of stars and galaxies. It is important that various methods do always overlap for a particular range of distances and determination of greater distances can be thus adjusted according the measurement of smaller distances. In this way, we gradually „climb up“to the remotest observed objects and we talk about the „cosmic distance ladder“; adapted from http://hetdex.org/images/dark_energy/scaling_the_universe_ladder_hr.jpg)
It may seem that the radius of the observable Universe would correspond to the value of 14 Gly. However, in 1929, Edwin Hubble discovered another crucial feature of our Universe – the space within has been expanding, the distances between galaxies have been increasing and for example, an object, that was in the distance of 13 Gly 13 billion years ago, is much farther today. That is why the radius of the observable Universe is estimated to be 45 Gly. (Fig.7).
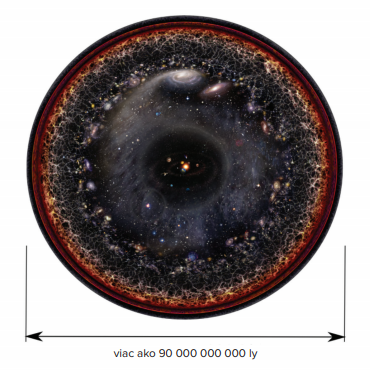
Fig. 7: An attempt at representation of the observable Universe; for us - observers, the Solar System is situated in an imaginary (and fictive) centre and in various distances we can observe various structures – objects of the Solar System, stars of the Milky Way, close and remote galaxies,germinal galaxies and ... „finally“a relic microwawe radiation from the times of approximately 400 000 years after the Big Bang. (source:Wikipédia)