INTRODUCTION
After the stage of the Main sequence the stars enter the final stages of their life. Depending on the mass, each star takes a different path. Until it finally ended up as a white dwarf, a neutron star or a black hole.
Stellar Evolution: Final stages
When the star has depleted all the hydrogen in its core, the nuclear rections stop. This takes away the source that maintains the equilibrium of the star. Its core starts to contract and become more and more hot. The outer layers, however start to cool and expand. The star transforms into a red giant.
The figure below shows a comparison of the present day size of the Sun (the small yellow dot in the white rectangle) and the size it will have in the red giant stage of its evolution – the difference is staggering!
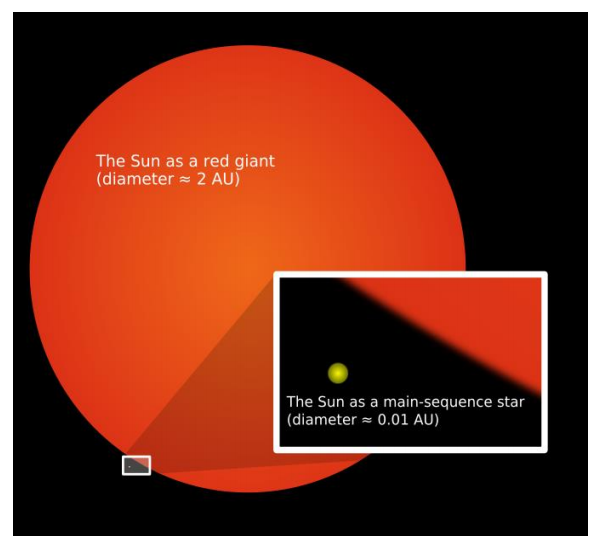
If the star is massive enough, the collapsing core can heat up enough to ignite another chain of nuclear reactions – the helium reactions in which helium is transformed into carbon and oxygen. The helium burning will maintain the equilibrium of the star. But these reactions will not last forever. In fact, they will deplete the helium in a relatively short time and the star will again become a red giant.
For stars, with masses up to about 4 times the mass to our Sun, after the helium is depleted, there will be no other source to maintain the equilibrium (no other nuclear reactions can be ignited in the core), so the core keeps collapsing until it shrinks down to about the size of the Earth, but with a much, much greater mass. A bit larger stars, with masses between 4 and 8 times the solar mass, the core can ignite another type of nuclear reactions – carbon ones, again fro a short time and then follow the fate of the less massive stars, but the remnant core will have a different chemical composition. The remnant core will continue to exist as a white dwarf.
The matter in the white dwarf is so tightly packed (has so high density), that a piece of it with the size of a mobile phone will weigh as much as an elephant on Earth!
White dwarfs, which are all about the size of the Earth, but have the mass of a star, puzzled the astronomers with their existence. Why has the collapse of the core stopped? What is the force that keeps the equilibrium? An answer to these questions is provided by quantum mechanics. The pressure of the fast moving electrons keeps the star from further collapse. The more massive the core of the initial star was, the denser the resulting white dwarf is. So the smaller the white dwarf’s size is, the higher its mass is. This paradoxical objects are in fact quite common in the Galaxy – there are plenty of stars, similar to the Sun and they all at some point will (or have already) become white dwarfs. The Sun will also become a white dwarf in another about 5 billion years. A famous white dwarf is the star Sirius B – the companion to the brightest star on our skies.
During the helium burning in the cores of the stars form a kind of heat pulses which spread outwards and “blow away” the outer layers of the stars in the surrounding space. These outer layers can contain more than half of the stellar mass. As a result around the star forms an expanding gaseous envelope that we call a planetary nebula. Planetary nebulae have nothing to do with planets – they just look like planets when seen through a small telescope.
On the figure below is sown a typical planetary nebula – M57, with a white dwarf at its centre. The planetry nebulae do not last long – they disperse in the interstellar medium for about 100 000 years, enriching it with heavier elements.
The stars that are more massive that about 8 solar masses can maintain their equilibrium a for bit longer, because their cores can heat up to higher temperatures and ignite more “exotic” nuclear reactions – like neon, oxygen and silicon, ending up with a core made of iron. When such a star is in the red giant, it is called red supergiant. The supergiant stage is followed by a huge, spectacular explosion, called supernova explosion. At this time a huge ammount of matter (the outer layers of the star) is blown out in space in the form of expanding gas envelopes, called supernova remnants.
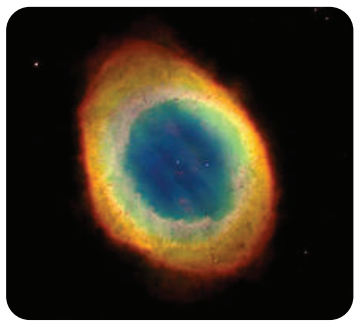
Below you can see an image in visible light of the remnant of the supernova, observed in the year 1054 – the famous Crab nebula in the constellation of Taurus.
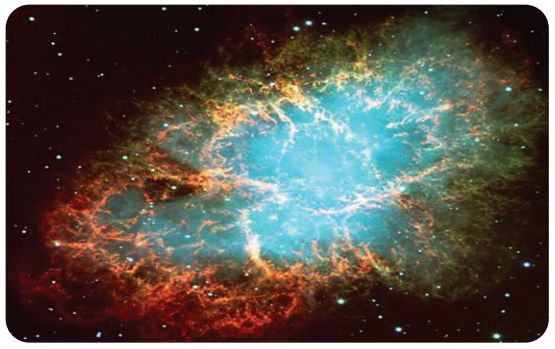
Because the exploding star was very massive, the remnant core was more massive than 1.4 solar masses and was not able to become white dwarf. Instead, since the electron pressure could not balance out the gravitation, it kept collapsing. During such a collapse not only the atoms, but also the nuclei of the atoms are destroyed and the resultant mass contains mostly neutrons. Now, the pressure of the neutron gas can stop the collapse and the remnant of the core becomes a neutron star. The density inside a neutron star is enormous – of the order of 1018 kg/m3 . The size, on the other hand, is tiny between 10 and 30 kilometers. This is the size of a large city. So imagine a couple of stars, the size of our Sun fit in the limits of, say London!
If the mass of the remnant exceeds about 3 solar masses, even the neutron gas pressure can not stop the collapse. The end result is what we call a black hole – an almost infinitely high density shrunk to and infinitely small size. Such objects have such an enormous gravitational force which bends space significantly and does not allow even light to escape it. So we can not really and directly see black holes. The only way to detect them is to observe the effects such objects have on the space and other objects around them – if there is matter from another close-by object spiraling down into them, it heats up considerably and emits in X-rays and gamma-rays, which we can detect. An example of such system is the X-ray source Cygnus X-1.
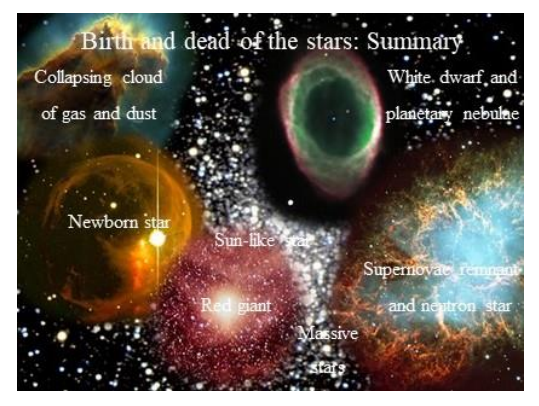